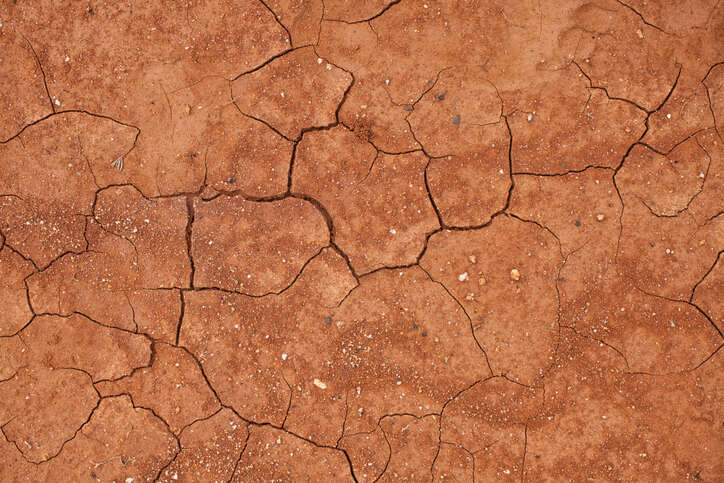
Alex Maltman investigates clay, a crucial wine soil type that is simultaneously familiar and mysterious.
Clay is familiar stuff. As children, we probably all took a lump of clay and modeled a little animal or something. We probably all know that it’s baked to make bricks and tiles, pots and plates. In fact, it’s a material used commercially in enormous quantities: in landfill linings, iron foundries, brake linings, explosives, drilling muds, animal housing, and so on, and so on.
And of course, its importance for wine can hardly be overstated, with its crucial influence on the nutritional status and drainage properties of vineyard soils. But also in the wine world, it’s used for such things as a sunscreen for vine foliage, clarifying wine, and for growing vine cuttings. Clay foundations can affect the very stability of winery buildings.
Even fermentation vessels, in recent years so fashionably made of oak or stainless steel, are for some producers now shifting back to the material used 8,000 years ago: clay.
Yet despite all this, it’s a rather mysterious substance.
What is it exactly? What is it made of? Where does it come from? And why does it behave in such unique ways?
In this article, I will discuss some aspects of this distinctive substance that are relevant to wine, and suggest that understanding just two fundamental attributes of clay goes a very long way toward explaining why it is so special.
Intricate architecture on a tiny scale
In order to understand clay, we have to peer down to the very atoms that make it. All the ground beneath our feet is made largely of just eight chemical elements bonded together; almost three quarters of it is oxygen and silicon, and most of the rest is aluminum, iron, calcium, magnesium, potassium, and sodium.
And the first three, together with some hydrogen to make hydroxide (OH), are principally what constitutes clay. In other words, clay is a common geological material, an earthy compound made primarily of silicon, oxygen, and aluminum.
But here’s the first of those two attributes. The elements are arranged in sheets. That sounds innocuous enough, but it really is one of the keys to why clay is so important. Sheets of inter-joined silicon and oxygen are stacked on top of each other and held together by the other elements in between to make a solid compound. Reflecting this sheet arrangement, each particle has an overall platy shape; an individual clay mineral is often pictured as a flake.
We can think of the sheets of atoms in a clay mineral as a multidecked sandwich, with the silicon and oxygen as the slices of bread and the other elements providing the fillings. And in the same way that sandwich fillings vary in how well they hold the bread slices together, so the silicon-oxygen sheets in clay vary in their inter-linking, some being weakly held together and others firmly bonded. It’s that simple, but I will come shortly to its crucial repercussions.
And the other fundamental feature of clay? Each flake is tiny, very tiny. An individual flake of clay mineral is at the very most 0.002mm (two thousandths of a millimeter) in length; most are even smaller. That’s roughly a hundredth of the thickness of a human hair, so obviously we can’t see the actual clay flakes—that requires advanced laboratory instruments.
Straightaway, we can see some consequences of this: Such tiny particles will easily clog the pores and conduits in soil, shutting down the drainage. And it’s why that lump of modeling clay is so malleable; it comprises innumerable, invisible tiny flakes that easily slip past each other such that the mass as a whole is easily molded.
It’s also why the very finest rock debris, with particles smaller than those in silt and much smaller than sand, is simply referred to as “clay.” It’s the main constituent of mud. So, we can think of clay as loose sediment, a soil, a lump, or even a weak rock, but those substances are all aggregates of countless, indiscernible tiny flakes of clay minerals.
Taking the sandwich analogy just one more step, a sandwich shop might arrange its products according to the nature of the bread—say, wholemeal, white, brown, and so on—and then by the different fillings. There could be quite a range, but of course they’re all sandwiches.
In the same way, mineralogists recognize different kinds of clay according to the nature of the sheets, the way they are stacked up, and the kinds and positions of the elements in between. This leads to a daunting number of different kinds of clay, different clay minerals, each with its own name and particular properties.
Here I will single out just a few examples from this extensive range for a closer look. First up, kaolin. It’s perhaps the most familiar type of clay, since it appears on the labels of everyday things such as pharmaceuticals (especially, these days, alternative medicines), cosmetics, and garden products.
A secret from the mystic East
Jean-Baptiste Darnet was a leading 18th-century surgeon in St-Yrieix, Haut-Vienne, France, so he always had to appear well turned out. He therefore appreciated the particularly crisp, clean laundry his wife always provided him with. And one day he happened to learn her laundering secret: She always added to the wash some of the white clay from the ground of their property.
Puzzled, the surgeon sent a sample of this intriguing clay to an apothecary friend for analysis, eventually to learn that he had stumbled across something very special, a kind of holy grail of the time. It was kaolin.
Ever since the porcelain figurines brought back from the East by early explorers like Marco Polo, Europeans had sought to replicate the fine product made in China. That porcelain had a purity of color, translucency, strength, and hardness far superior to the soft pottery being made in Europe at the time, at places such as Sèvres, near Paris. However, the materials used in China were jealously guarded; they were a mystery in Europe.
Eventually, however, in 1712, the Jesuit missionary Francis d’Entrecolles managed to hoodwink some samples of the secret raw substance and get them back to France. There they were eagerly analyzed and the results published, which immediately prompted a hunt for a Western source of similar material.
In England, Josiah Wedgwood became interested, and he even attempted to import and test the fabled “white burning clay” of Cherokee tribes living deep in the wilds of the southern Appalachians. But M Darnet had found a supply right in his garden in France. Other sources were soon discovered, and before long a new European industry was born.
By the end of the century, the clay at St-Yrieix was supplying the factories that were starting up at nearby Limoges and was being sent to Sèvres and as far as Copenhagen. Around the same time, similar clay was found at places like Meissen in Germany, and at Cornwall in England, much to the delight of Mr Wedgwood, and to Mr Spode, Mr Beswick, Mr Doulton, and the rest.
But all these clayey materials retained the name of the place in China from which Père d’Entrecolles had procured his original samples, the village of Kaulin, about 28 miles (45km) northeast of Ching-teh-chien, in Jiangxi province.
An alternative transcription of the name is Kaolin, and this version was to become the widely adopted name for the clay. The European porcelain made from the clay became universally known simply as “china.”
Kaolin is so suited to this use partly because it consists of—as clay minerals go—relatively large, rigid flakes. Its chemical composition is also relatively simple, with just aluminum and hydroxyl (OH) in addition to the silicon and oxygen.
The resulting bonding is strong, with none of the complications that arise in other clays that involve various metals. The bonds are strengthened further on firing, to produce the thin, strong, translucent sheets characteristic of fine china.
In practice, there are usually various impurities present in kaolin, and certainly there are in so-called kaolin vineyard soils; geologists refer to the pure mineral as kaolinite.
We can use kaolin for a glimpse of how clay comes about in nature, in the earth. Clay minerals are not primary constituents of rocks but are what geologists call “secondary,” forming at or near the ground surface where ground- and rainwaters act on the existing materials. A good example is the rock granite, the major mineral of which is feldspar, rich in potassium and aluminum.
Water in the ground reacts chemically to replace the feldspar with a potassium-rich clay mineral (called sericite), which then reacts further to lose its potassium, leaving kaolin. In fact, kaolin soils are found most commonly on granite bedrock and typically still contain a lot of potassium.
Vineyard examples are the soils at Temecula Valley in Southern California, Spain’s Rías Baixas, and Portugal’s Dão. At Larnage, in the Crozes-Hermitage region of France, the thick kaolin soils derived from the granite of the northern Rhône not only lead to acclaimed Syrah wines but are quarried for the famous Le Panyol bread and pizza ovens.
While the relatively large clay flakes of kaolin usually give soils of adequate drainage, their chemical simplicity can lead to nutritional problems. The absence of calcium and carbonate gives acid soils that can restrict nutrient availability and curb root development.
This is the case in, for example, South Africa’s Western Cape, where kaolin soils are common, derived from granites such as those at Darling, Paarl, and Stellenbosch. Down on the Cape peninsula, just south of the historic Constantia and Steenberg wine estates, there are old kaolin quarries at Noordhoek and Fishhoek. The vineyard soils there reach acidities with a pH lower than 4, and growers routinely have to incorporate lime in the soils in order to achieve a balanced nutrient availability.
The other issue is the typically high potassium content arising from the clays associated with kaolin. Where the vine’s uptake-selectivity mechanisms are unable to curb the abundant potassium, the excess tends to react preferentially with the wine’s tartaric acid.
The resulting potassium tartrate is poorly soluble and precipitates, forming the well-known white crystals, stained purple in the case of red wines, seen at the bottom of some bottles and corks. These “wine diamonds” are themselves innocuous enough, but the resulting lowering of acidity can lead to flabby, unstable, and short-lived wines.
There has been considerable excitement in recent years about a new use of kaolin in vineyards. High levels of ultraviolet radiation can disturb vine growth and sunburn grapes; parts of Australia such as the Granite Belt of Queensland can lose 15 percent of a crop due to sunburn.
Sunscreen treatments in the past have had mixed results, but the new approach uses nanotechnology and involves spraying comminuted kaolin particles, each of which is smaller than a billionth of a meter. Tests have suggested that this not only blocks the ultraviolet radiation but that the whitened leaves increase light reflectance into the canopy and improve evenness of grape ripening.
Moreover, it seems that nano-sprayed vines repel insect pests, including the sharpshooters that spread the dreaded Pierce’s disease. At the same time, it seems the vines optimize the way they use available water. Because around two thirds of the world’s grapevines are currently cultivated in semi-arid conditions, this use of nano-kaolin could become increasingly valuable in these times of rising temperatures and concerns about water sustainability.
The miracle of montmorillonite
Moving on to the family of clay minerals known as montmorillonite—and also as smectite—we jump in complexity and difficulty. But we certainly must take a look, because it’s a clay that is vital in many vineyards and can be important in the winery itself, in ways nicely illustrating the significance of those two properties fundamental to all kinds of clay.
Most montmorillonite originates from the weathering of igneous rocks like basalt and related volcanic deposits. For example, the young volcanic rocks of the Tokaj-Hegyalja region of Hungary are currently weathering into montmorillonite, which, in addition to underlying some of the best vineyard sites, is quarried for industrial use.
Geological processes can rework such volcanic material and re-deposit it elsewhere as sediments, so areas of sedimentary rock such as shale can also yield soils rich in montmorillonite; it’s a reasonably common clay in vineyards around the world.
Now for those two properties—and first, the size of montmorillonite flakes. They are much, much smaller than kaolin: a single flake is little more than a millionth of a millimeter in thickness. (That, for instance, is 50 times smaller than a single Ebola virus). The length can be anywhere between 30 and 300 times more than the thickness, so the particles are distinctly flaky in shape.
A major consequence of all this, however, is not so much the unimaginable minuteness but the surface area each flake presents. The figures are staggering. Just a single gram of montmorillonite, with its myriad constituent minuscule flakes, will contain within it a clay surface area greater than a couple of parking spaces.
And here’s the thing: No mineral is perfectly formed; there are always some unbonded atoms left spare at the surface, and these can be calcium, magnesium, iron, and so on—the very elements that vines need as nutrients.
With most minerals, the number of these spare atoms is trivial, but with montmorillonite its colossal surface area means that there are a great many of them, and being loosely held they are available to be detached and used by the vine roots.
This is fundamentally where the nutrients required by the vine come from. Mycorrhizae may be involved, and the soil humus provides nitrogen and phosphorus and recycles nutrients, but the detachable atoms at the surface of montmorillonite and similar clays are the underlying key to the fertility status of the soil.
The second critical aspect is the behavior of montmorillonite with water, and here it’s that sheet structure again. Water can seep between the sheets, prising them apart, to be held there without actually bonding with the clay structure. Substantial amounts of water can be stored, and also loosely adsorbed onto the surfaces of the clay flakes. The benefits are obvious for vineyards on free-draining soils or prone to drought. Here are some illustrations.
The Pomerol region is famous for its clay, but even so, there are considerable spreads of sand and gravel where soil drainage can be excessive, especially on more elevated ground. And the highest point is occupied by Petrus.
At that celebrated site, however, within reach of the vine roots, is a montmorillonite clay capable of storing water from the winter rains. In fact, the same clay comes and goes across northeast Pomerol, over a comma-shaped area that includes a clutch of top-notch châteaux, such as Trotanoy, Le Pin, Vieux Château Certan, and L’Evangile, together with St-Emilion’s Cheval Blanc and Figeac.
All of them attach great importance to the presence of this montmorillonite (known locally as argile bleue) in their soils, at least for some of their vines.
Unlikely though it often seems to Westerners, fine wine is made in a number of Middle Eastern countries, and Jordan is one case in point. Originally, Omar Zumot used his French training to make good wines southwest of the country’s capital, Amman, but he was always searching for some optimal site.
Eventually, he found it up near the border with Syria, in a region known as the Mafraq. It’s one of the most fertile parts of Jordan because of the basalt that once flowed here from the now-extinct Jabal Druze volcano to the north.
But at an elevated place called Samas Al-Sarhan, the basalt is weathering to montmorillonite clay, reducing the soil fertility nicely for vines and storing soil moisture from the winter rains. Thanks to this clay, with minimal irrigation even in this arid area, Zumot’s St George wines now rank among the finest in the Middle East.
In recent years, a number of commentators have tipped Romania as a country to watch for promising wines, with the Dealul Mare region, just north of Bucharest, as probably the best. So, around the villages of Finţești, Urlaţi, and Fantanele, for example, several vineyards are attracting attention, and they all have a certain amount of montmorillonite clay.
Mihail Rotenberg was attracted to the village of Ceptura in this area to produce his eponymous wines, especially Merlot, when he realized that the soil was similar to that in parts of St-Emilion—that is, underlain by montmorillonite. The area receives limited rainfall, and there are some worries that the soil is too fertile, so the patches with montmorillonite soil are the most desirable.
There are snags to all this, however. Water entering the clay and prising apart the internal sheets causes the flake to swell in size: Sodium montmorillonite expands to several times its original size. The swollen clay particles readily coalesce to produce a sticky mass, blocking the channels that allow water movement in the soils and hence closing down the drainage.
Obviously, the facility for storing water is of little use if the water can’t get out. In addition, rainwater may not be able to penetrate down into this impermeable soil, leading to surface waterlogging and undesirable puddles in the vineyard.
On top of this, there’s the converse problem that when the clayey soil eventually dries out, it shrinks and cracks. The soil becomes denser, hindering root growth, and the vines respond by trying to ripen fruit prematurely.
The problem is illustrated at the Cristom vineyards in Oregon’s Eola-Amity Hills AVA. The winemaker, Steve Doerner, has always specialized in producing Pinot Noir, but he’s found that Pinot Gris copes better in the block that has montmorillonite. Even so, though he had to install irrigation in order to help manage the late-season water supply, he still usually ends up picking here first.
Because of their behavior with water, montmorillonite and related clays are sometimes referred to as “shrink-swell” or, alternatively, “heave and subsidence” clays, and the latter phrase illustrates a further problem.
If water seeps beneath a building during wet times, any shrink-swell clays in the foundations will expand and try to heave the building up, albeit probably no more than an apparently trivial few millimeters. When the clay dries out, the building settles back down, but only for the whole process to start up again in the next rainy season.
Eventually, after several seasons of this repeated lifting and settling, cracks may begin to appear in the walls, and the structure may begin to founder. A common response is simply to fill in the cracks, but that is merely masking the symptoms. To resolve the problem, either some flexibility has to be introduced into the building structure or the construction must be underpinned to new foundations.
The phenomenon may be insidious, but it’s a very costly business. Parts of France have suffered dramatically in recent years from flooding events, but the resulting financial losses are less than those arising every year from heave and subsidence.
In the United States, the costs of dealing with swelling clays on average exceeds those arising from headline-making disasters such as tornadoes, earthquakes, and hurricanes. In the UK, heave and subsidence accounts for the single biggest year-on-year insurance claim for building damage—around £500 million a year. The clays, incidentally, are most common in the east and southeast of the country, exactly where vineyard expansion is currently taking place.
Furthermore, the problem is worsening. Swelling clays are rarely mentioned in discussions of climate change, but according to a recent report, they pose the greatest potential risk to critical infrastructure, such as highways, telecoms, water, gas, and electricity distribution. And all this is because the clay atoms are arranged in sheets.
A fine story from the wild west
One of the two senators elected in 1820 to represent Missouri, the year it became a state, was Thomas Hart Benton. A rich and colorful political career was to follow, but his overriding endeavor throughout was to promote the continued westward expansion of the United States, to meet its “manifest destiny.”
Thus, he helped author the first Homestead Act and encouraged the founding of the state of Oregon and the annexation of the Republic of Texas. He also championed the rights of the American Fur Company to deal in Montana, even when the operation was accused of being more interested in trading whiskey than furs.
This resolute support from a politician 1,500 miles (2,400km) away was acknowledged by the Fur Company in 1846, when it completed construction in Montana of a new outpost on the uppermost reaches of the Missouri River. They named it in his honor: Fort Benton.
Shortly after that, two pioneering geologists of the American west found, close to the new fort, layers of volcanic ash, now largely converted to clay. They later noted that the same ashy rocks extended way south, far into Wyoming, but they decided to name the entire formation after the site of their original discovery and therefore termed it the Fort Benton Group.
Actually, down in Rock Creek, Wyoming—five saloons but no churches, and an official population of 91 including a “tramp”—a certain William Taylor was already digging out a “soapy clay” from these very rocks and transporting it all the way to a cosmetics company in way-off Pittsburgh.
And by the end of the century, the state geologist of Wyoming, Wilbur C Wright, was confirming that Taylor’s clay indeed had special properties—so much so that he felt it justified its own name. He called it bentonite. Today, you can see sacks of bentonite in wineries all around the world.
The monetary value of bentonite to the wine industry is now huge, but it’s just a fraction of its total commercial worth. Last year, more than 2 million tons of the stuff was mined in 47 different countries, with a value approaching $2 billion.
Nearly half is used in iron foundries (as a high-temperature bonding for molding sand) and in pet-waste absorbents. Among numerous other things, it’s used in pet foodstuffs and, curiously, even somehow finds its way into human food. William Taylor could hardly have imagined!
We now know that bentonites around the world vary somewhat in the geological minerals they contain but that one of them always dominates and accounts for those special properties—montmorillonite. And once again, it’s that sheet structure that’s important.
One use of bentonite is as a soil conditioner, with its montmorillonite increasing the water-holding abilities of soils and giving them weight. The moistened clay has the effect of “thickening” the soil, giving it body and making it “heavier.” And stickier.
Anyone who has tramped a vineyard with montmorillonite soils—Vaillons in Chablis, say—in the rain will know how eagerly the sticky clumps cling onto your boots (just as in the hell of the Flanders trenches during World War I; 90 percent of the Ypres clay is montmorillonite).
The main use of bentonite in the wine industry, however, is for clarification, in the process known as fining. Just as montmorillonite expands with soil water, so it swells when mixed with wine and attracts proteins and flecks of solids, the things that can cause wine to remain hazy rather than settling clear.
It can also remove excessive tannins and improve the wine’s mouthfeel. In a typical procedure, at the end of fermentation, bentonite granules are stirred with water and the resulting slurry added to the wine.
The thin suspension adsorbs any potentially haze-forming substances and slowly settles to form a sludge, from which the wine can be racked, now star-bright as consumers expect. Some winemakers filter the wine in addition to, or instead of, fining, but filtering doesn’t remove any soluble compounds that may later go on to cloud the wine.
The procedure of fining has its critics, however, both as a matter of principle—interfering with the natural progression of the wine—and through it possibly removing desirable aromatic compounds.
Also, a study at UC Davis showed that aluminum was stripped from the bentonite during fining, particularly by high-acid wines, leading to what is regarded by some as contamination and, arguably, to possible health issues. (Incidentally, this raises questions about the new fashion for packaging wine in aluminum cans.)
The use of bentonite in vinification has taken on a new significance in recent years, with the explosion of enthusiasm for veganism. We increasingly see on wine labels phrases like “suitable for vegetarians and vegans,” which often prompts the question, “Aren’t all wines, being fermented fruit, suitable?”
Well, no, and the main reason lies in some wines being fined by using animal-derived agents such as casein (milk protein), chitin (from crustacean shells), albumen (from egg whites), gelatin (from boiling animal parts), and isinglass (from fish bladder membranes). Consequently, vegan suitability comes about either through the wine not having been fined or because a non-animal product, of which bentonite is the most common, has been employed.
It’s perhaps somewhat ironic that biodynamic wines, with their aura of extreme closeness to nature, aren’t suitable for vegans, because of their prescribed dependence on animal products—cow horns stuffed with cow manure, for instance.
And as a final twist, back in Rock River, Wyoming, which first saw the commercial extraction of the bentonite now crucial to vegan wine drinkers, the clay is no longer extracted; the business is long gone. Instead, these days Rock River’s main commercial product is… beef.
Back to the nursery
You probably know vermiculite, perhaps as the stuff sold in garden centers for rooting seedlings or from the heat insulation in your loft, though you’ve probably not thought of it as a clay. But it is, occurring in the ground just like other clays, though in this case heavily processed.
It involves magnesium, and sometimes iron, and so tends to form by the weathering of magnesium-bearing minerals such as those found in volcanic rocks. It also contains water.
Unlike, however, the loosely attached liquid water that participates in the kinds of shrinking and swelling we’ve just discussed, in vermiculite this water is bonded wholly within the solid crystal lattice; its chemical formula is written to include H2O.
Apparently, it was known long ago in Japan that this kind of clay could be heated to produce weird little shapes and provide amusement for children. Then, in 1824, almost certainly the same kind of stuff was dug by Thomas H Webb from the ground near Worcester, Massachusetts.
He wrote that “if subjected to the flame of a blowpipe or that of a common lamp, it expands and shoots out into a variety of fanciful forms, resembling most generally small worms having the vermicular motion exact.” So, he proposed to “term it vermiculite (worm breeder) from vermiculare, to breed or produce worms.”
Vermiculite was born, though the material remained something of a curiosity until the early years of last century. In 1923, the first commercial mine was started in Libby, Montana, to supply the construction industry.
Today, the clay is mined all over the world and industrially processed. In particular, the extracted material is cleaned and then heated very quickly to force the internal water to turn to steam, which blows apart the mineral sheets to give the now-familiar accordion-like form.
Having been expanded in this way, the clay is clean and light to handle, odorless, mold resistant, and has a remarkably low density that makes it great for heat and noise insulation.
And for those same reasons it makes an ideal material for embryo plant roots, a “rooting medium” in horticultural speak. Thus gardeners can buy bags of it for raising plant cuttings, and if you’ve ever bought already established plants in little pots, you might have noticed bits in the soil sparkling in the sunlight—they’re vermiculite.
The kinds of temperatures employed in its production—upward of 212°F (100°C)—mean that situation has no direct relevance to vineyards, but all vine nurseries employ expanded vermiculite (or another mineral product called perlite).
Generating new vines is an aspect most of us rarely think about, but these days it’s a vital and highly specialized business. The latest technologies involve sophisticated in vitro micro-propagation of vines, but vermiculite is still required to bring on the seedlings before planting out in the vineyard.
So, we’ve seen the importance of clay in the wine world, as well as glimpsing some of the wide range of industrial applications. But clay might also be of relevance to you as an individual, seeing as—we are told by promoters—applying or ingesting clay “fuels creative passion,” gives hair “a less-defined, sleeker finish,” “promotes normal stools,” “detoxes armpits,” and so on.
It’s quite an impressive range. One advertisement for a facial treatment based on clay declares that “you can’t buy happiness, but you can buy clay, and that’s pretty much the same thing.” Clay is certainly wonderful stuff.